description:
Evidence for the birth of the universe is raining down on you. It’s called the cosmic microwave background, and it’s had quite a journey. Born in the stupendous annihilation of matter and antimatter seconds after the big bang, trapped in the hot plasma of the expanding universe for 380,000 years, and then suddenly released when the universe cooled to the point that atoms could form, this echo of creation has been on an uninterrupted voyage through space for 13.7 billion years—until it reached you. The cosmic microwave background is just one of the many clues about the history and nature of our universe that make the science of cosmology a wondrous, fascinating, and philosophically profound field of study.
episodes:
The first of 10 lectures on the physical sciences covers Einstein’s special and general theories of relativity, which undermined 200 years of physics and launched a wholly unexpected revision in our conception of the universe.
The universe is lumpy at the scale of galaxies and galaxy clusters. But at larger scales it seems to be smooth and similar in all directions. This property of homogeneity and isotropy is called the cosmological principle.
Picking up the story of quantum theory in the 1920s, this lecture covers its growth into a mature system called quantum mechanics through key contributions by Niels Bohr, Erwin Schrödinger, and Werner Heisenberg.
Take a quick trip through the history of the universe, from the big bang to a conjectured future of ever-accelerating expansion. Eight key stages are in between, including the condensation of atoms, the birth of the first stars, and the formation of galaxies.
Astronomers use a “distance ladder” of overlapping techniques to determine distances in the universe. Triangulation works for nearby stars. For progressively farther objects, observers use pulsating stars, the rotation of galaxies, and a special class of supernova explosions.
Measurements of the Doppler shift of galaxies in the early 20th century revealed that essentially all were moving away from us. The more distant ones are moving faster, in direct proportion to their distance. This is the famous Hubble Law, which implies an initial “big bang.”
Defining distances in cosmology is tricky, since an object’s distance continually increases with cosmic expansion. There are three important distances to consider: the emission distance, when the light set out; the current distance, when the light arrives; and the distance the light has traveled.
Atomic matter comprises less than 5% of the total cosmic census. This realization came with the discovery of dark matter (23%) in the 1970s and dark energy (73%) in the late 1990s. They are “dark” because they are detectable only by their subtle gravitational effects.
Einstein’s theory of gravity suggests that space could be positively or negatively curved, so that giant billion-light-year triangles might have angles that don’t add up to 180°. This lecture discusses the success at measuring the curvature of the universe in 1998.
Has the universe’s rate of expansion always been the same? You answer this question by applying Newton’s law of gravity to an expanding sphere of matter, finding that the expansion was faster in the past and slows down over time.
You investigate why the three great eras of cosmic history—radiation, matter, and dark energy—have three characteristic kinds of expansion. These are rapid deceleration, modest deceleration, and exponential acceleration. The last is propelled by dark energy, which makes the universe fall outward.
By looking sufficiently far away, and hence back in time, we can witness the “flash” from the big bang itself. This arrives from all directions as a feeble glow of microwave radiation called the cosmic microwave background (CMB), discovered by chance in 1964.
You visit the million-year-old universe to take in the sights: a slowly changing rainbow sky, a low-density super-hot atmosphere, and everywhere, brilliant light.
Earth’s atmosphere is the medium for sound waves. Was the primordial atmosphere of the young universe also conducive to sound? The answer is yes, as proved by tiny variations in the CMB. You hear re-creations of this primordial sound, which is somewhere between a musical note and noise.
Slight differences in the properties of the universe lead to slightly different sound spectra. These differences allow cosmologists to use sound spectra to determine what kind of universe we inhabit. You listen to the primordial sound of three universes of different densities.
There are two key players in the growth of structure in the universe: atomic matter and dark matter. This lecture looks at how dark matter created the framework into which atomic matter ultimately gathered. From these concentrations of atomic matter, stars and galaxies eventually formed.
How does the universe move from a smooth, uniformly filled state, ringing with primordial sound, to one with stars and galaxies? You follow the process that resulted in the first stars, which were massive, brilliant beacons lighting up the early universe.
Once the first generation of stars had formed, they began to collect into infant galaxies—chaotic collections of stars and gas that were much smaller than today’s huge, majestic galaxies. The best telescopic images, such as the Hubble Ultra Deep Field, show this exuberant period.
The young universe matured into its present adult phase through the ceaseless collision of small galaxies to make big ones. Our own Milky Way galaxy formed this way and has a major collision looming in its future—3 or 4 billion years from now.
At the heart of essentially every galaxy lies a giant black hole, a million to a billion times as massive as the sun. These monsters play an important role in the lives of galaxies. Confirming their existence was an impressive achievement of the Hubble Space Telescope.
A simulated intergalactic trip shows you the three-dimensional distribution of galaxies in our region of the universe. On the largest scale, galaxies form a weblike pattern that matches the peaks and troughs of the primordial sound in the early universe.
Humans are no less a part of the universe than any star or galaxy, and the atoms in us can only have arrived via a cosmological route. This lecture discusses how heavier elements came from stellar processes, including nuclear reactions and supernova explosions.
The theory of atom genesis in the interiors of stars is confirmed by the proportions of each element throughout the cosmos. The relative abundances hardly vary from place to place, so that gold isn’t rare just on earth, it’s rare everywhere.
There is one glaring error in the story of atom-genesis inside stars: far too much helium. It must have been produced in the big bang along with other light elements. According to theory, this occurred when the universe was just three minutes old.
This lecture reviews the six key pieces of evidence that indicate that we live in an expanding, evolving universe that began in a hot big bang a few billion years ago. Together with five key observational datasets, these pieces yield a remarkably consistent picture called the concordance model.
This lecture begins your investigation of the universe during its first second, which is an immense tract of time in nature. To understand what happened, you need to know how nature behaves at ultrahigh energy and density. Fortunately, the physics is much simpler than you might think.
At one microsecond, all the particles of the standard model of particle physics were present. By the time the universe was a few minutes old, matter-antimatter annihilation and nuclear fusion had roughly created today’s composition of mainly photons and a few protons, helium nuclei, and electrons.
You venture into the bizarre world of the opening nanosecond. There are two primary themes: the birth of matter and the birth of forces. Near one nanosecond, the universe was filled with a dense broth of the most elementary particles. As temperatures dropped, particles began to form.
Although the standard big bang theory was amazingly successful, it couldn’t explain several fundamental properties of the universe: Its geometry is Euclidean, it’s smooth on the largest scales, and it was born slightly lumpy on smaller scales. The theory of cosmic inflation offers a comprehensive solution.
This lecture shows how the early universe might enter a brief phase of exponentially accelerating expansion, or inflation, providing a mechanism to launch the standard hot big bang universe. This picture also solves the flatness, horizon, and monopole problems that plagued the standard big-bang theory.
Inflation also helps explain the origin of cosmic lumpiness. During inflation, tiny quantum mechanical fluctuations can get frozen as real fluctuations when they expand faster than light, away from their place of origin. The expansion then stretches these fluctuations to cosmic proportions.
All the matter and energy in stars and galaxies is exactly balanced by all the negative energy stored in the gravitational fields between the galaxies. Inflation is the mechanism that takes nothing and makes a universe—not just our universe, but potentially many.
Why does the universe have the properties it does and not some different set of laws? One approach is to see the laws as inevitable if life ever evolves to ask such questions. This position is called the anthropic argument, and its validity is hotly debated.
The next 20 years in cosmology promise to be no less interesting than the last. New experiments are in the works to deepen our understanding in six areas: dark matter, dark energy, the universe’s first billion years, galaxy evolution, inflation, and the early universe.
Why is it possible for us to understand the universe? Could our apparent comprehension be an illusion? There are good reasons to believe that we have authentic access to the deepest secrets of nature and that we should not be surprised to feel at home in the universe.
SIMILAR TITLES:
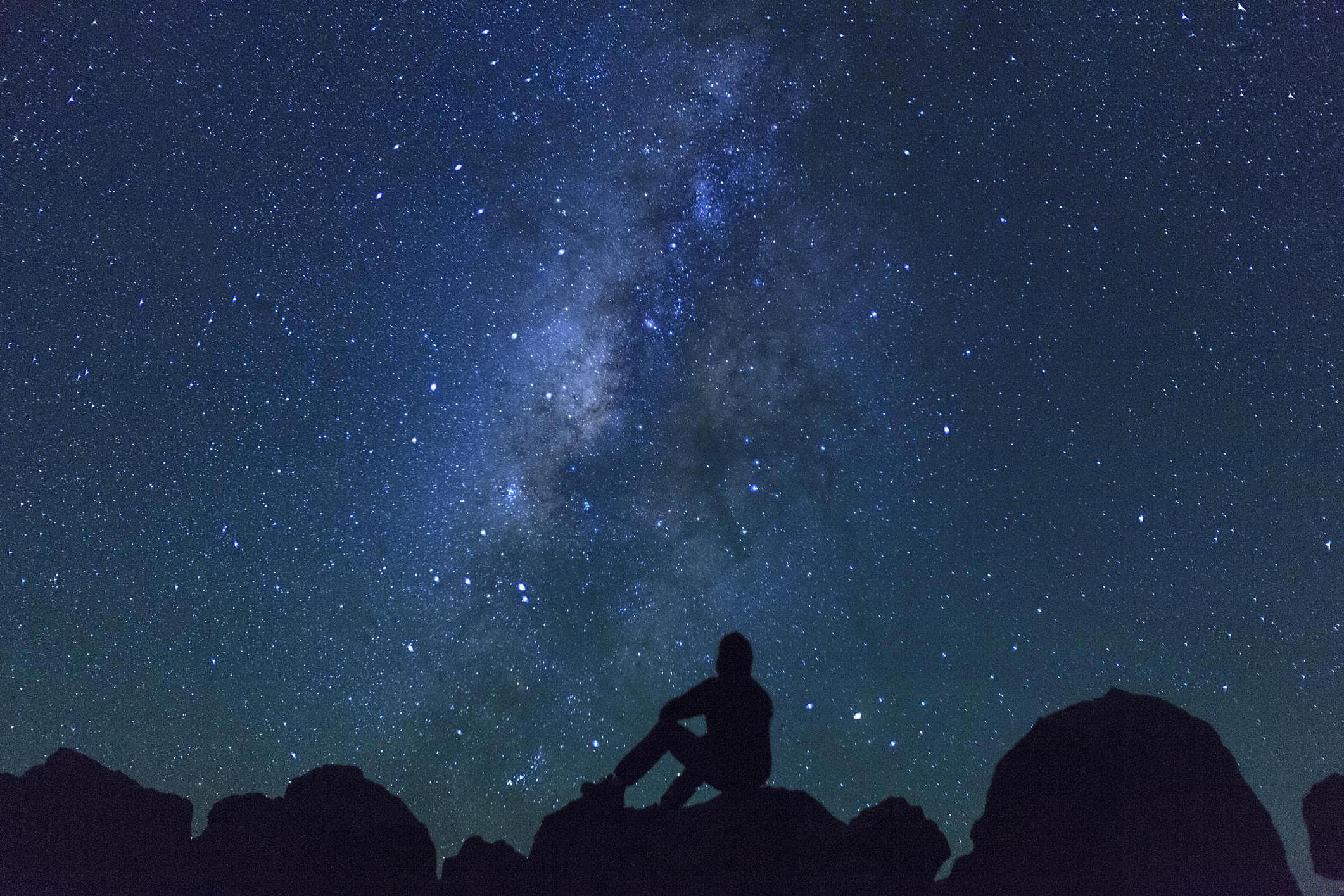
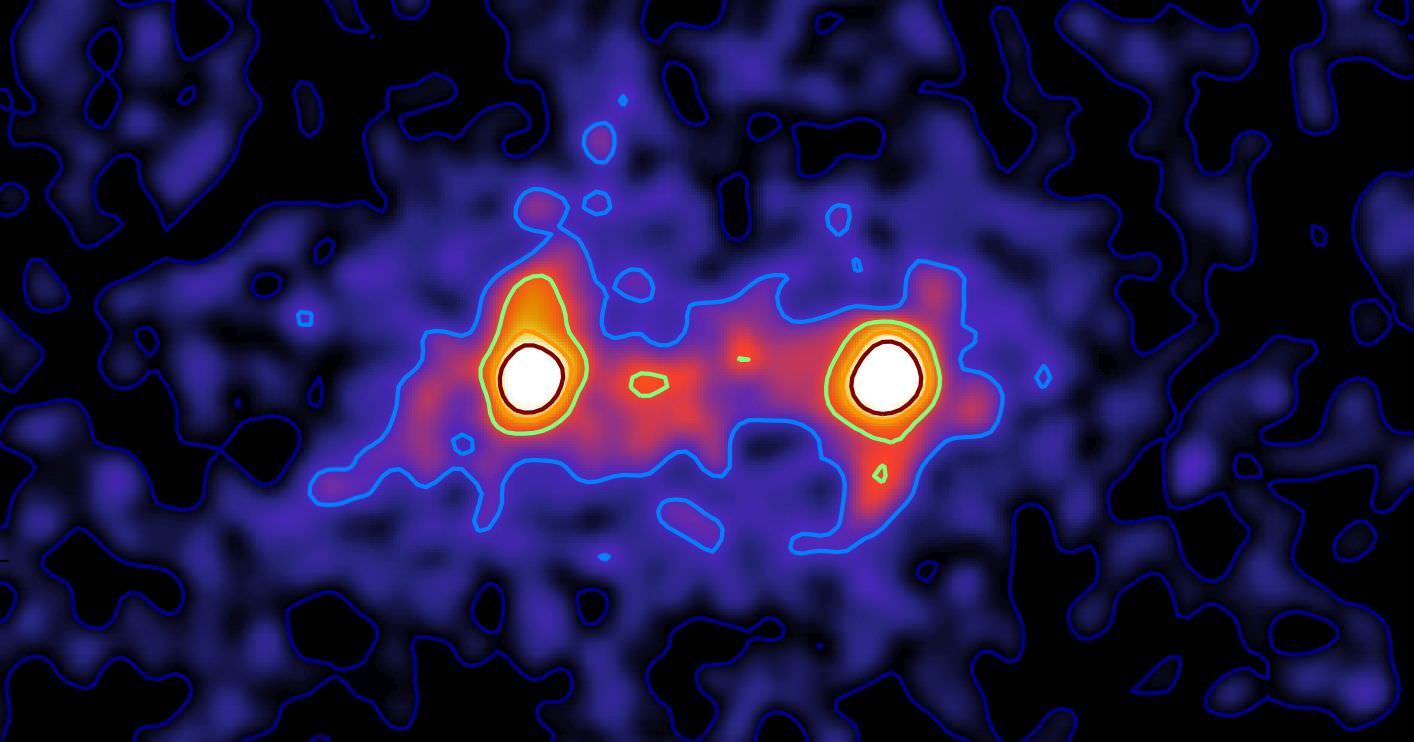
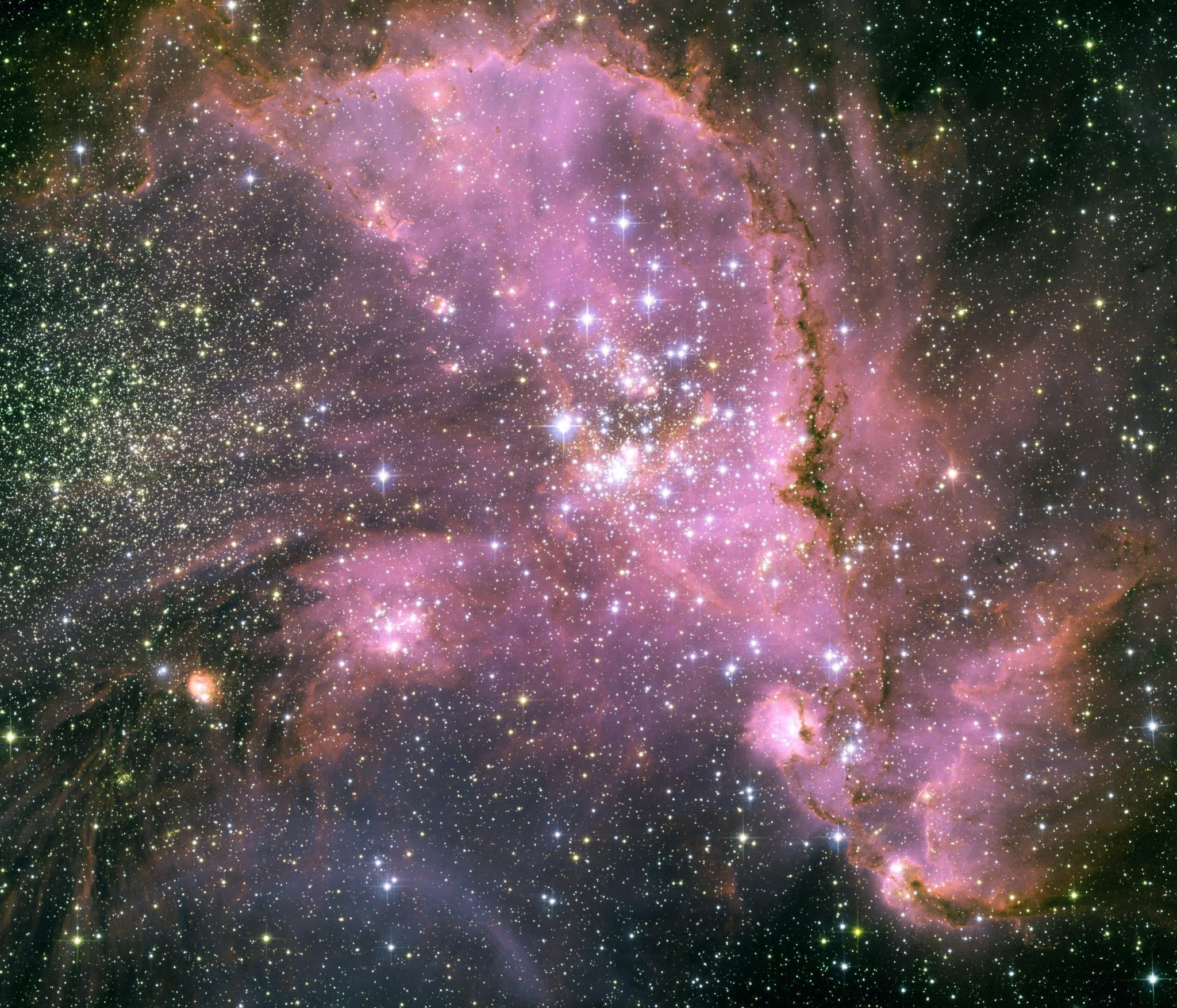
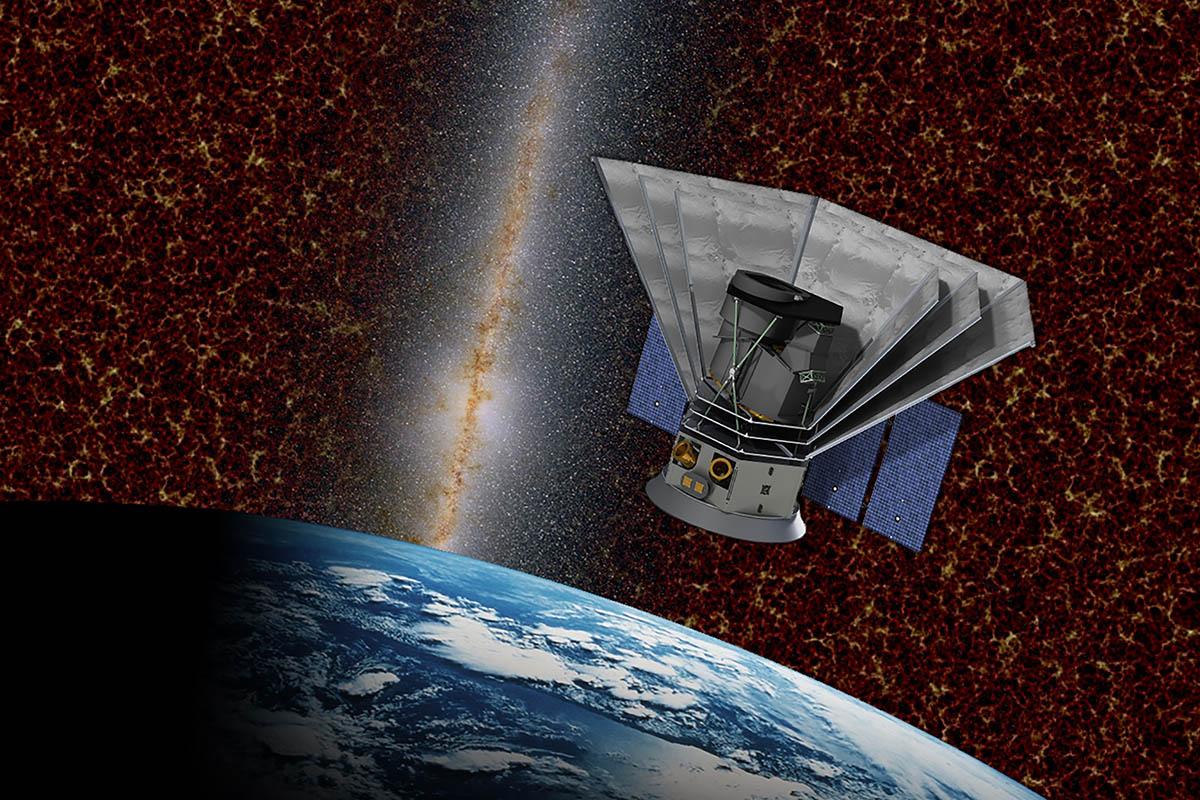
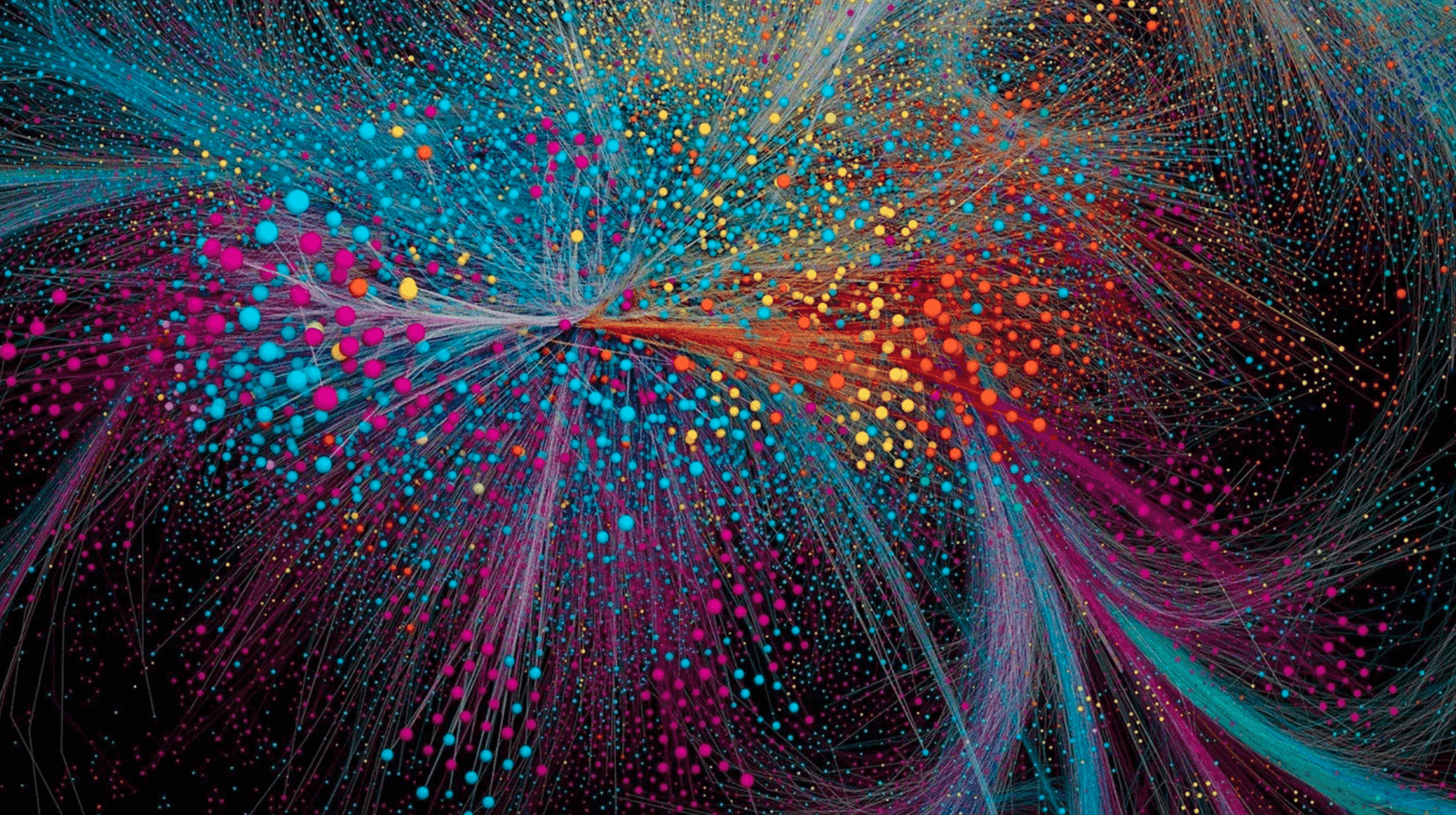
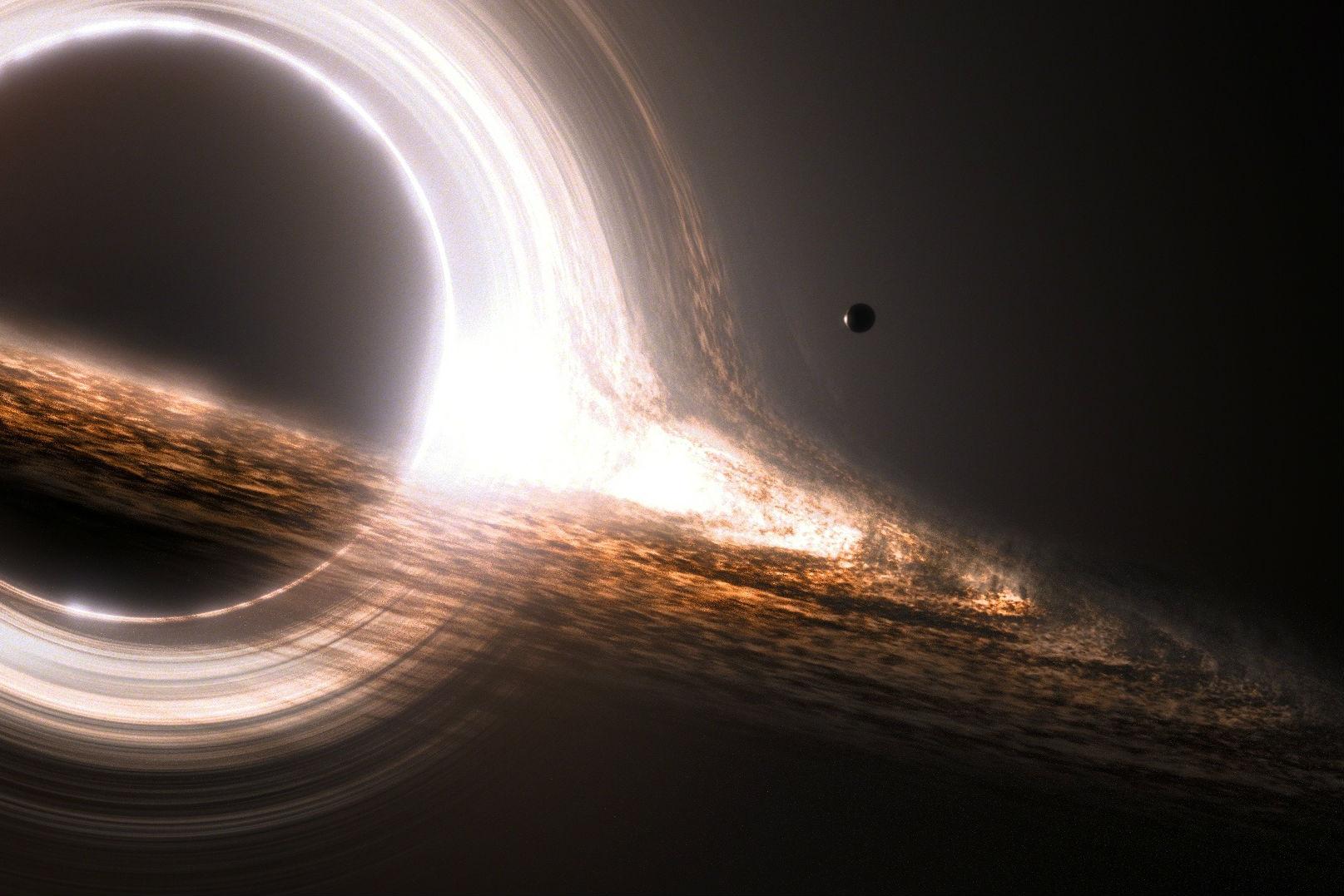